Classical and quantum simulations with ultracold fermionic mixtures in optical lattices
Ultracold quantum gases provide a unique opportunity for quantum simulations of interacting many-body systems. Tremendous progress in experimental techniques over the last years has allowed to control all the important aspects of such simulations and thus, has offered insights into physical mechanisms that eluded understanding in conventional condensed matter systems. The full understanding of experiments is not possible without reliable theoretical description.
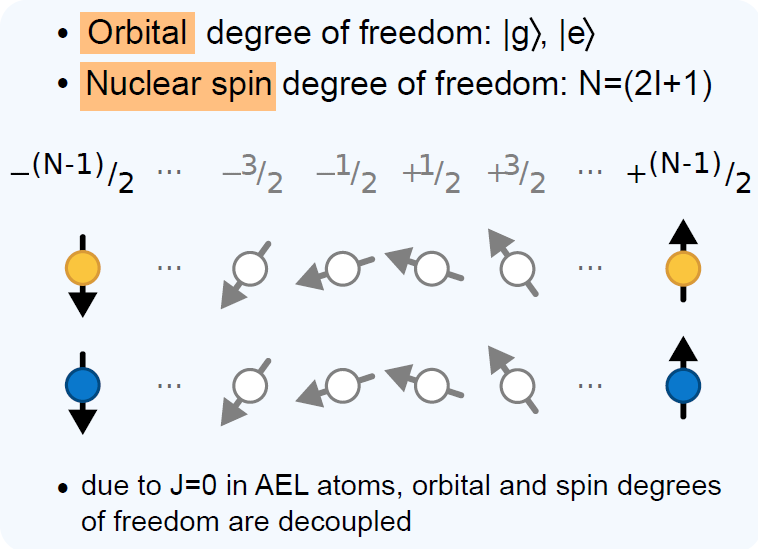
Alkaline-Earth-Like (AEL) Atoms as good candidates for the realization of the solid state systems.
Our research is motivated by recent experimental developments with ultracold gases of alkaline-earth-like (AEL) atoms, which offer many advantages over the more commonly used alkalimetal atoms. AEL atoms, in particular, the fermionic isotopes of 87Sr and 173Yb, set truly new perspectives for investigation of new states of matter. The unique properties of these atoms give an exclusive opportunity to study Hamiltonians beyond the reach of alkali-based experiments, such as the two-band Hubbard model, the Kugel-Khomskii model originally proposed for transition-metal oxides, the Kondo lattice model studied in the context of manganese oxide perovskites and heavy fermion materials, and SU(N)-symmetric magnetic systems. Ytterbium is particularly versatile due to its large number of bosonic and fermionic isotopes with a wide range of interaction strengths and also has some advantages, such as low-lying (meta)stable excited electronic states, decoupling of the nuclear spin from the electronic degrees of freedom, and different ac polarizabilities of the ground and metastable states. The main goal of our reasearch is to investigate the phenomenon of orbitalmagnetism within the two-band Hubbard model (2BHM) and to determine parameter regimes that are most favorable for orbital and magnetic phases, such that they can be observed within ultracold atomic gases in optical lattices at empirically accessible values of temperatures and entropies, using the experimentally relevant setups of the 2BHM. The realization of the studies leads to a better understanding of magnetism in ultracold multi-component quantum gases. It allows to determine optimal values of doping for observing magnetically and orbitally ordered phases in ultracold gases. It explains some existing experimental results and also guides further experimental research by providing reliable theoretical predictions.
- A. Cichy, A. Ptok,
Superfluidity of fermionic pairs in a harmonic trap. Comparative studies: local density approximation and Bogoliubov-de Gennes solutions,
J. Phys. Commun. 4 (2020) 055006 - A. Cichy, K. J. Kapcia, A. Ptok,
Phase separations induced by a trapping potential in one-dimensional fermionic system as a source of core-shell structures,
Scientific Reports 9 (2019) 6719 - A. Cichy, A. Ptok,
Reentrant Fulde-Ferrell-Larkin-Ovchinnikov superfluidity in the honeycomb lattice,
Phys. Rev. A 97 (2018) 053619 - A. Ptok, A. Cichy, K. Rodriguez, K. J. Kapcia,
Critical behaviour in one dimension: unconventional pairing, phase separation, BECBCS crossover and magnetic Lifshitz transition,
Phys. Rev. A 95 (2017) 033613 - A. Cichy, K. Cichy, T. P. Polak,
Competition between Abelian and Zeeman magnetic field effects in a two dimensional ultracold gas of fermions,
Annals of Physics 354 (2015) 89-100 - A. Cichy, R. Micnas,
The spin-imbalanced attractive Hubbard model in d = 3: Phase diagrams and BCS–BEC crossover at low filling,
Annals of Physics 347 (2014) 207-249.
The main aim of our research is to provide theoretical description for novel ultracold atomic gases experiments, in close coordination with them. In other words, we perform classical simulations of phenomena occurring in quantum simulators, to achieve full understanding of a broad class of interacting many-body systems. The main research hypothesis is that the investigated class of phenomena is crucial from the point of view of generic mechanisms of unconventional, including high-temperature, superconductivity and superfluidity as well as quantum magnetism. By isolating the relevant mechanisms in a fully controlled way, this will shed new light on the most important problems of condensed matter physics.