Phononics
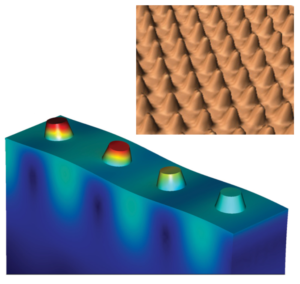
The sound waves we encounter in our everyday life are elastic (acoustic) waves with macroscopic wavelengths measured in tens of centimetres. Their wavelengths can be dragged into the nanoscale by enhancing the frequencies to GHz range. High-frequency elastic waves can be processed in nanodevices using typical wave phenomena such as interference or refraction. The various forms of the elastic waves can be generated by the interplay between the anisotropy of elastic properties of the medium and the directional character of mechanical oscillations.
Phononic crystals are unique and promising materials for controlling and manipulating the propagation of elastic waves. The periodic nature of such materials gives them novel properties that cannot be found in bulk materials, such as near-zero group velocity or formation of bandgap – the range of frequency where the acoustic waves cannot propagate. Defect-free phononic crystals are beneficial in nanoscale fabrication because they can significantly reduce time-consuming technological processes while potentially improving device precision. The introduction of defects into phononic crystals (for example, local irregularities in the phononic lattice) paves the way to creating new devices for controlling acoustic wave transmission. Implementing an additional layer to a phononic structure fragment enables a bandgap to evolve and the vibrations of the pillars to be localized. The modulation of elastic properties and the dimension of nanostructures provide additional opportunities to influence wave propagation. Besides that, phononic crystals and their applications, such as communication, sensing, and optomechanics, have recently received much attention.
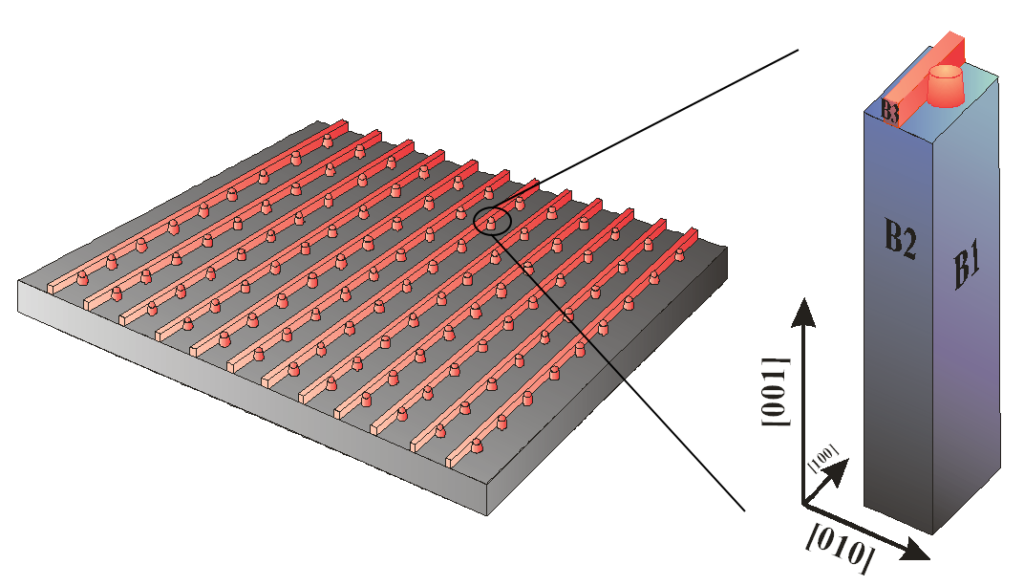
The propagation of surface acoustic waves (SAW) in a nano-engineered phononic system composed of one-dimensional (1D) and two-dimensional (2D) phononic structures, each with a unique location that defines its bandgap. Using the same material to create two structures of different dimensions in a single technological process appears to be another positive step toward expanding the application possibilities of phononic materials.
We used non-destructive testing methods such as Brillouin scattering of light to determine the SAW dispersion relation for our research. The propagation of surface acoustic waves along the silicon surfaces covered with a metallic nanostructure was studied using a six-pass, tandem Brillouin spectrometer (JRSandercock Scientific Instruments), which ensures a contrast of 1015.
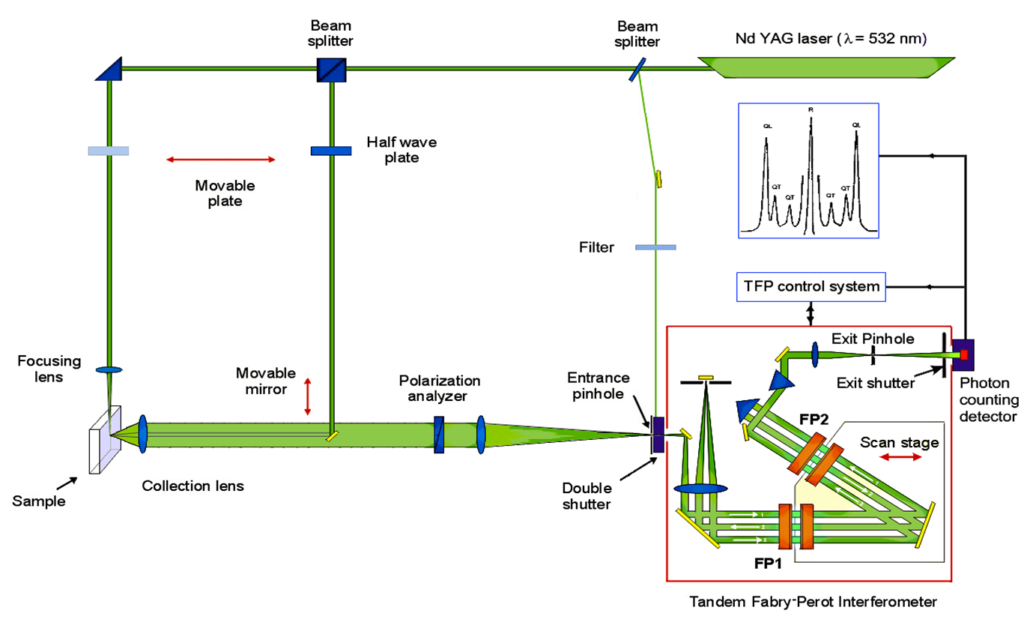
The finite element method (FEM) was used to perform the numerical simulation of the anticipated dispersion relation. After extending the measuring system with various magnetic fields, we measure the dispersion dependencies of magnonic structures in various geometrical configurations of the wave vector and the direction of the external magnetic field. After that, the measurements concentrated on magnetoelastic interactions in an extensive multilayer structure based on ferromagnetic materials.
- N.K.P. Babu, A. Trzaskowska, S. Mielcarek, H. Głowiński, O. M. Chumak, M. Zdunek, J. W. Kłos, J.W., M. Krawczyk, Interaction between Thermal Magnons and Phonons in a CoFeB/Au Multilayer, IEEE Magn. Lett. 10,8886514 (2019). DOI:10.1109/LMAG.2019.2950304
- J. Kharlan, P. Bondarenko, M. Krawczyk, O. Salyuk, E. Tartakovskaya, A. Trzaskowska, V. Golub, Standing spin waves in perpendicularly magnetized triangular dots, Phys. Rev. B 100,184416 (2019). DOI:10.1103/PhysRevB.100.184416
- M. Zdunek, A. Trzaskowska, J. W. Kłos, N.K.P. Babu, S. Mielcarek, Investigation of phonons and magnons in [Ni80Fe20/Au/Co/Au]10 multilayers J. Magn. Magn. Mater. 500, 166428 (2020). DOI:10.1016/j.jmmm.2020.166428
- A. Trzaskowska, P. Hakonen, M. Wiesner, S. Mielcarek, Generation of a mode in phononic crystal based on 1D/2D structures, Ultrasonics 106,106146 (2020). DOI:10.1016/j.ultras.2020.106146
- A. Trzaskowska, B. Mroz, Surface phonons in topological insulator Bi2Te3 investigated by Brillouin light scattering, Sci. Rep. 10,11812 (2020). DOI:10.1038/s41598-020-68690-z
- N.K.P. Babu, A. Trzaskowska, P. Graczyk, G. Centała, S. Mieszczak, H. Głowiński, M. Zdunek, S. Mielcarek, J.W. Kłos, The Interaction between Surface Acoustic Waves and Spin Waves: The Role of Anisotropy and Spatial Profiles of the Modes, Nano Lett. 21, 946 (2021). DOI:10.1021/acs.nanolett.0c03692